Molecular Motors & the Cytoskeleton
29/09/14 14:53
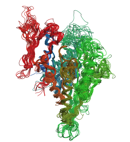
A principal aim in the study of molecular motors, is a detailed understanding of how chemical energy, derived from the hydrolysis of ATP, is converted into mechanical energy. This process, termed mechanochemical transduction, is thought to involve the amplification of small nucleotide induced conformational changes into larger movements that ultimately drive cellular motility.
We have performed a number of studies on kinesin and myosin motors to probe possible mechanisms for conformational change. These studies have used available sequence and crystal structure data, complemented by structural bioinformatics approaches on motor homologues and analogues (which provide key landmarks in conformational analyses). The simulations are based on an empirically derived potential energy function for the description of inter/intra-molecular interactions. Methods employed include normal mode analysis (for intrinsic mechanical properties); equilibrium and non-equilibrium accelerated molecular dynamics (for the response of systems to changes in the nucleotide state), and electrostatic and Brownian dynamics simulations (for diffusional encounters of the motor with its respective track).
<< Back to Research Overview